Share a link
An Introduction to Immunofluorescence
Advances in fluorophore chemistry and instrumentation mean many different types of immunoassays now employ a fluorescent readout. But, for reliable results, immunofluorescent experiments must be carefully optimized.
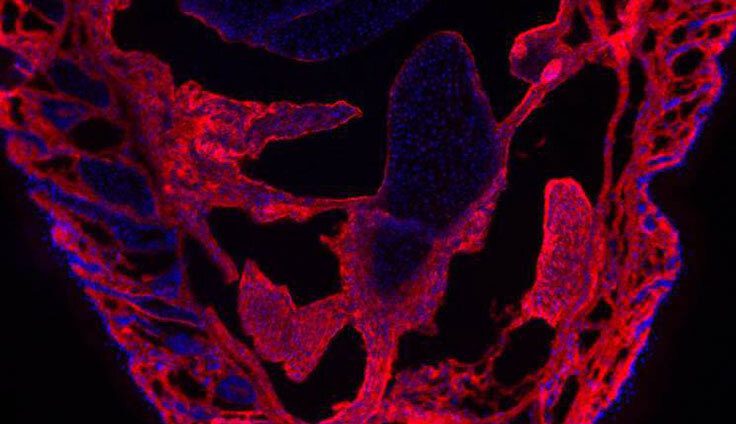
What is immunofluorescence?
Immunofluorescence is a term that describes the use of fluorophore-labeled antibodies for detecting analytes of interest. Following antibody binding to the target, light of a specific wavelength is used to excite the attached fluorophore, which then emits light at another, longer wavelength for measurement. Both primary and secondary antibodies can be labeled with fluorophores, enabling either direct or indirect detection. Additionally, other fluorophore-labeled reagents (e.g., streptavidin) can be incorporated into immunostaining protocols to provide signal amplification.
We offer an extensive selection of fluorophore-labeled streptavidin reagents, which include streptavidin conjugated to FITC, TRITC, PE, and various tandem dyes.
What is the difference between direct and indirect immunofluorescence?
Direct immunofluorescence uses fluorophore-labeled primary antibodies for detecting analytes of interest. An advantage of this approach is that it shortens the experimental workflow by removing the need for a secondary antibody incubation step and any associated washes. In addition, direct immunofluorescence allows for using several primary antibodies from the same host species for multiplexed experiments (provided the primary antibodies have proven specificity for their antigenic targets) since there is no risk of secondary antibody cross-reactivities.
Indirect immunofluorescence instead uses a combination of unlabeled primary antibodies and fluorophore-labeled secondary antibodies for detection. It is more common than direct immunofluorescence due to the broad range of secondary antibody reagents that is available commercially. A main advantage of indirect immunofluorescence is that it provides signal amplification as a result of multiple secondary antibodies binding to each primary antibody. Importantly, this can improve the detection of low abundance antigens.
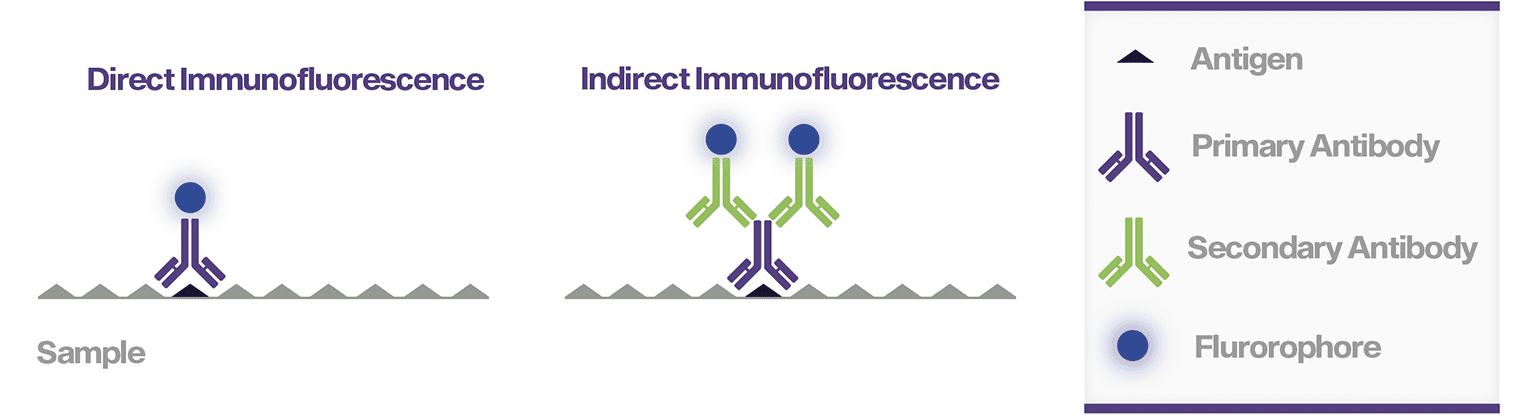
What are the different types of fluorophores in immunofluorescence imaging?
Fluorophores comprise naturally-occurring fluorescent proteins and synthetic dyes. Examples of the former include the algal proteins R-phycoerythrin (PE) and allophycocyanin (APC), while synthetic dyes include fluorescein isothiocyanate (FITC), tetramethylrhodamine (TRITC), the Alexa Fluor® dyes, the cyanine dyes, and Texas Red®, to name but a few. Complementing these products, tandem dyes consist of two covalently linked fluorescent molecules - a donor and an acceptor - which produce a measurable readout through Förster resonance energy transfer (FRET).
Our product portfolio encompasses almost 20 different fluorophore options, including tandem dyes, to increase flexibility for experimental design.
What distinguishes different fluorophores?
Fluorophores are defined by several innate characteristics. The most well-known are the excitation and emission maxima, which are the wavelengths at which light is maximally absorbed and emitted, respectively. Deriving from these, the Stokes shift represents the difference between the two maxima and can be exploited for increased flexibility in multi-color panel design. Other important fluorophore properties include brightness and photostability; the latter impacts fluorophore performance over time, which can be compromised by photobleaching (a form of light-induced fluorophore degradation).
Fluoromount-G® Anti-Fade is a water-soluble compound designed to prevent photobleaching in microscopy applications and can be used with frozen and paraffin sections, whole mounts, and cells.
What types of experiments have an immunofluorescent readout?
Almost any immunoassay format can be adapted to produce a fluorescent readout. While immunocytochemistry (ICC) and flow cytometry have become synonymous with fluorescence, immunohistochemistry (IHC), Western blotting, fluorescent-linked immunosorbent assay (FLISA), and various bead-based immunoassays also use fluorescent detection.
How can I optimize my immunofluorescence experiment?
Fluorophores present unique challenges when it comes to optimization. Critically, sources of autofluorescence must be addressed and spectral overlap avoided to ensure reliable results.
Handle samples carefully to avoid causing autofluorescence
Dead cells, cellular debris, growth media components (e.g., serum), and aldehyde fixatives (e.g., paraformaldehyde) can all cause autofluorescence and yield false positive results. Strategies to minimize autofluorescence include harvesting cultured cells at an appropriate (healthy) density; avoiding harsh centrifugations, vortexing, or freeze-thawing; incorporating sufficient wash steps into protocols; and analyzing aldehyde-treated cells within 24 hours of fixation. It is also important to keep sample material at 4oC or on ice.
Match fluorophores to instrumentation
Ensuring fluorophores are compatible with the platform that will be used for detection is vital. Excitation and emission maxima should be matched to the instrument's lasers and detectors, respectively, which can easily be checked in the instrument manufacturer's handbook. Spectra viewers are useful open-access tools that can help with assessing whether a particular fluorophore is suitable for use.
Pair fluorophore brightness with target abundance
Understanding where, when, and how intensely targets of interest are expressed is essential for fluorophore selection. It is recommended that bright fluorophores (e.g., AF647, PE, APC) be used for detecting low abundance analytes, and vice versa, and it is worth referring to published data and online resources such as UniProt, PAXdb, and proteinatlas.org for information about expected protein expression levels in different sample types.
Take steps to avoid spectral overlap
Where a panel of different fluorophores will be used for interrogating multiple targets simultaneously, fluorescence spillover (the detection of one fluorophore in the channel of another) can cause unwanted background signal. To minimize this risk, it is important to identify fluorophores with non-overlapping spectra and to spread these across as many lasers and detectors as possible. This process can be simplified using online panel builder tools to guide fluorophore selection and panel design.
Include relevant controls
While proper controls underpin the success of any experiment, workflows employing an immunofluorescent readout also require that controls account for sources of unexpected fluorescence. These should include unstained samples to determine levels of background fluorescence; isotype controls to confirm that any observed signal is due to primary antibody specificity for the antigen; and secondary antibody-only controls to determine whether non-specific binding of secondary antibody reagents is an issue. Flow cytometry experiments should additionally incorporate fluorescence minus one (FMO) controls for evaluating fluorescence spread and compensation controls to address spillover.
Our product portfolio contains over 250 isotype controls, for hosts including mouse, rat, human, goat, and rabbit.